Helicobacter pylori is a bacterium linked to serious gastrointestinal diseases, including gastric cancer. This article delves into its impact, transmission routes, and innovative solutions like those from the NIAGARA project to ensure safer drinking water worldwide.
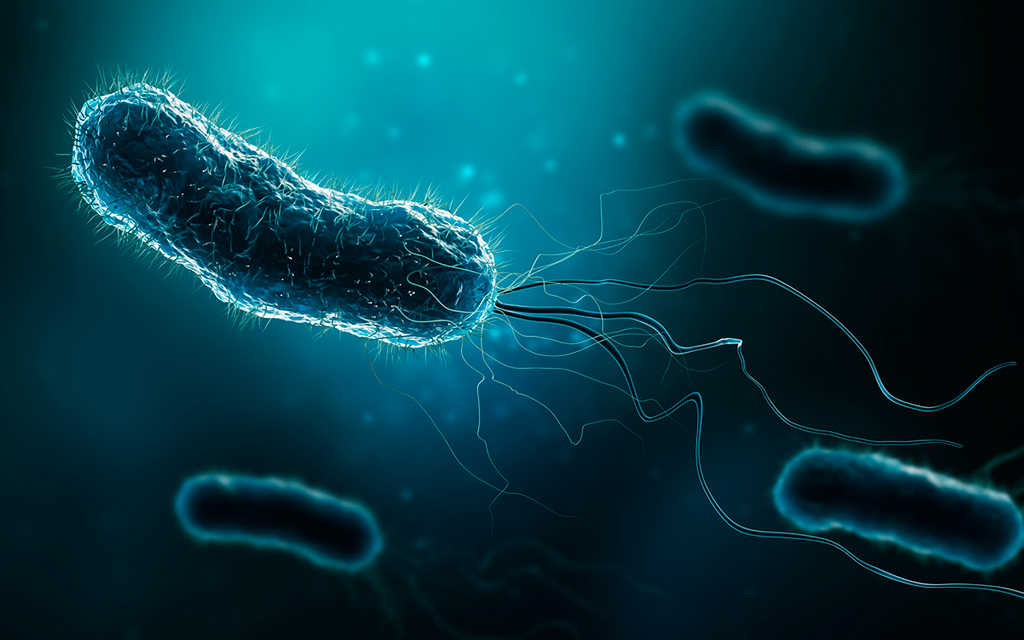
Helicobacter pylori (H. pylori) is a gram-negative bacterium that is closely related to various gastrointestinal diseases, including gastritis, gastric ulcers, and gastric cancer (Sharndama and Mba, 2022). Recognized as a Class I carcinogen by the World Health Organization (WHO) in 2017, this bacterium’s persistence and resilience have made it a significant public health concern.
What is the global prevalence of H. pylori infection?
H. pylori infections are prevalent worldwide, but infection rates vary significantly depending on geographical location and socio-economic conditions. In developing countries, the infection rate can reach as high as 85%-95%, whereas, in developed nations, it is significantly lower, ranging between 30%-50%. This stark contrast is largely attributed to factors such as healthcare accessibility, sanitation standards, and population density (Khoder et al., 2019). According to recent data, infection rates are highest in Africa, followed by Asia and Europe, with lower rates in the Americas and Oceania. (Liu et al., 2024).
What are the transmission routes of H. pylori?
H. pylori infection can occur through multiple transmission routes, which include person-to-person contact, animal-to-human transmission, and contamination through food and water. The most common transmission occurs through close contact, particularly within families, where maternal-child transmission is often observed (Yang et al., 2023). Recent studies have also indicated that animals, particularly livestock, may serve as vectors for H. pylori. In rural regions of Poland, shepherds and their families showed a significantly higher prevalence of H. pylori compared to farmers not exposed to sheep (Papież et al., 2003; Soloski et al., 2022). H. pylori can also be contracted from contaminated water and food sources. The bacterium can survive in water sources such as lakes, rivers, and even groundwater, often through fecal contamination (Duan et al., 2023).
H. pylori and Drinking Water Quality
Water safety is a critical issue when it comes to preventing H. pylori infection. In developing countries, poor sanitation and untreated water sources such as wells and springs are major contributors to the spread of this bacterium. In contrast, in developed countries, drinking water disinfection processes may fail to eradicate pathogens such as H. pylori, which are resistant to common disinfection methods like chlorination.
Innovative Solutions: The NIAGARA Project
The NIAGARA project aims to address the challenge of H. pylori contamination in drinking water by developing innovative solutions to detect and remove this pathogen. A core component of this project is the design of a multi-analyte biosensor for routine water quality monitoring. These biosensors will detect H. pylori and other key water pollutants, such as BPA, imazalil, and pharmaceuticals like ibuprofen. The project is also developing an immobilised enzymatic degradation system to efficiently remove chemical pollutants and pathogens such as H. pylori from drinking water. Additionally, the project will explore UV/TiO2 photocatalysis as a potential disinfection method, capable of inactivating a range of harmful pathogens, including H. pylori.
The need for effective and sustainable water treatments to address water pollutants such as H. pylori contamination in drinking water is urgent. With the increasing global prevalence of this pathogen, especially in regions with inadequate water treatment, it is essential to adopt new, advanced technologies that can detect and eliminate H. pylori and other harmful pollutants. By implementing advanced detection and treatment technologies, we can significantly reduce waterborne infections, protect public health, and move closer to a future with safe, clean drinking water for all.
Learn more and stay up-dated about our on-going efforts to combat drinking water pollution through the NIAGARA project in our social media LinkedIN and X.
References
Duan, M., Li, Y., Liu, J., Zhang, W., Dong, Y., Han, Z., et al. (2023). Transmission routes and patterns of helicobacter pylori. Helicobacter 28:e12945. doi: 10.1111/hel.12945
Khoder, G., Muhammad, J. S., Mahmoud, I., Soliman, S. S. M., and Burucoa, C. (2019). Prevalence of Helicobacter pylori and its associated factors among healthy asymptomatic residents in the United Arab Emirates. Pathogens 8:44. doi: 10.3390/pathogens8020044
Liu M, Gao H, Miao J, Zhang Z, Zheng L, Li F, Zhou S, Zhang Z, Li S, Liu H and Sun J (2024) Helicobacter pylori infection in humans and phytotherapy, probiotics, and emerging therapeutic interventions: a review. Front. Microbiol. 14:1330029. doi: 10.3389/fmicb.2023.1330029
Papież, D., Konturek, P. C., Bielanski, W., Plonka, M., Dobrzanska, M., Kaminska, A., et al. (2003). Prevalence of Helicobacter pylori infection in polish shepherds and their families. Dig. Liver Dis. 35, 10–15. doi: 10.1016/s1590-8658(02)00004-x
Sharndama, H. C., and Mba, I. E. (2022). Helicobacter pylori: an up-to-date overview on the virulence and pathogenesis mechanisms. Braz. J. Microbiol. 53, 33–50. doi: 10.1007/s42770-021-00675-0
Soloski, M. J., Poulain, M., and Pes, G. M. (2022). Does the trained immune system play an important role in the extreme longevity that is seen in the Sardinian blue zone? Front Aging 3:1069415. doi: 10.3389/fragi.2022.1069415
Yang, T., Zhang, Y., Zhang, H., Wu, X., Sun, J., Hua, D., et al. (2023). Intracellular presence and genetic relationship of Helicobacter pylori within neonates’ fecal yeasts and their mothers’ vaginal yeasts. Yeast 40, 401–413. doi: 10.1002/yea.3891